OUR RESEARCH
Soft Condensed Matter Physics
Soft condensed matter physics (also known as complex fluids) studies systems that are mechanically soft such as colloids, emulsions, surfactants, polymers, liquid crystals, and various biomaterials including DNA and proteins. These materials display a broad range of microstructures and phase behaviors and have a myriad of applications in material, food, paint, and cosmetic industries and in medical technology. This is a relatively new area of study in physics and thus presents new opportunities and challenges in developing interesting new concepts and novel experimental techniques and theoretical models. Because of its overlapping with many different disciplines, the study of soft matters offers promising new developments in physics, chemistry, chemical engineering, material sciences, biology, and environmental science. Our research is concerned mainly with the study of fundamental issues related to the soft matter physics using modern experimental methods of condensed matter physics. We are interested in inter-particle forces, microstructures, diffusion, aggregation and other dynamic processes in colloidal suspensions and polymer solutions and in their mixtures; structures and dynamics of a monolayer of colloidal particles at liquid-liquid interfaces; non-equilibrium processes in suspensions and at liquid-solid interfaces; sedimentation of non-Brownian particles in simple and complex fluids; microrheological properties of polymer solutions, gels, and biomaterials; anomalous diffusion of membrane-bound proteins and lipids in living cells. Small-angle neutron scattering, laser light scattering, atomic force microscopy, optical and florescent microscopy, and multi-particle tracking are used in the study of complex fluid systems.
1. Particle sedimentation and non-equilibrium pattern formation in suspensions of non-Brownian particles
Flows of solid particles suspended in a continuous liquid are encountered in a wide range of industrial processes, such as in chemical, metallurgical, plastics, pharmaceutical, and food processing. Because of their fundamental importance and wide applications, the particulate two-phase flows have become an active research area in soft matter physics. In recent years, much attention has been focused on the study of the microstructures formed in the particulate flows. These microstructures strongly influence the macroscopic rheological properties of the suspension and affect various material processes. In a recent experiment, we find band and other pattern formation in a settling suspension of uniform size and density, non-Brownian particles completely filling a horizontal rotating cylinder. The system shows a series of sharp pattern changes that are mapped out as a function of the rotation period and suspension viscosity. The experiment demonstrated that a large number of patterns and rich dynamics result from interplay between the gravitational and centripetal forces. Similar patterns are also observed in binary granular mixtures contained within a horizontal rotating cylinder, but in our system there is no sliding granular surface responsible for pattern formation. Unlike the granular system, however, we believe that the continuity equation and Navier-Stokes equation for a compressible fluid are sufficient to describe the phenomenon in terms of continuum mechanics. Another experiment we are working on is the study of sedimentation of heavy particles under gravity through a quiescent fluid. The main issue in particle sedimentation is to understand how hydrodynamic interactions created by the motion of many surrounding particles in the fluid affect a test particle's mean settling velocity and its variance at different particle concentrations. Because of the long-range hydrodynamic interactions, simple theoretical calculations as well as computer simulations have indicated that the velocity variance might diverge with increasing sample size. Experiments, however, find no size dependence for the particle velocity fluctuations. Solving the divergence problem, therefore, has become a profound issue in the study of particle sedimentation. In a recent experiment, we measured particle configurations in sedimentation using a direct imaging method. The experiment showed that number fluctuations of the setting particles are no longer proportional to the mean particle number and are suppressed significantly with time on large length scales. These findings are consistent with our recent proposal that the convective response of the suspension to ordinary number fluctuations reduces them; bring the suspension to a marginally stable state, in which the statistics are such that unstable convection is minimized.
2. Microrheological properties of complex fluids
A focused area of the current research in complex fluids is the study of the microrheological properties of polymer solutions, gels, and large biomolecules, such as DNA and polymeric actin, when the relevant length scales become comparable to the molecular dimensions. For example, the cytoplasmic transport is known to be influenced strongly by the mechanical properties of the cytoskeleton network at submicron scales. Our recent work focused on a simple problem of the transport of probe particles in semi-dilute polymer solutions. With a well-characterized colloid-polymer mixture, we varied the microscopic interaction between the colloidal particle and the polymer chain and measured its effect on the particle motion by dynamic light scattering and ultracentrifugation. Because the basic molecular interactions are chosen to be simple, we were able to explain the observed three different behaviors of the particle motion in terms of the microstructures formed in the colloid-polymer mixture. The experiment therefore resolved a longstanding issue in the field. A natural extension of the experiment is to study the local viscoelastic properties of polymer gels and biomaterials using dynamic light scattering and particle tracking techniques together with optical microscopy. We are now developing a new light scattering technique to measure the viscoelastic response between two probe particles separated by a small distance. This technique will provide a useful tool for the study of the microrheological properties of biomaterials.
3. Long-ranged attraction between charged colloidal particles at aqueous interfaces
Attraction between colloids of same charge is a controversial issue in colloid science and biology and has been with us for many years. Understanding of such attraction is extremely important as it determines the stability and structure formation of a variety of macromolecules in aqueous solutions, including proteins and DNA. An example of “like-charge" attractions is that between the charged polymer latex spheres when they are dispersed at an aqueous interface (say, at the water-air interface). Despite intensive theoretical investigations over the past decade, a satisfying explanation for the origin of the attraction still remains illusive. The lack of progress is partially due to a lack of well-controlled experimental studies of particle interactions at the interface. Recently, we have carried out an optical and atomic force microscopic (AFM) study of interactions between charged polystyrene spheres at a water-air interface. Optical observations of bonded particle clusters and formation of circular chain-like structures at the interface demonstrate that the interaction potential is of dipole origin. AFM phase images show patchy domains on the colloidal surface, indicating that the surface charge distribution is not uniform as is commonly believed. Such surface heterogeneity introduces in-plane dipoles, leading to an attraction at short inter-particle distances. The experiment thus is a breakthrough in the mechanism for like-charge attractions at the interface, which also provides new insights into the general understanding of like-charge attractions occurring in other colloidal systems.
4. Development of an atomic-force-microscope-based hanging-fiber resonator for the study of interfacial hydrodynamics and wetting dynamics
Recently, we developed a new capillary force apparatus using atomic force microscope (AFM) as a force sensor. As shown in the figure below, the probe used in the experiment contains a long vertical glass fiber with one end glued onto an AFM cantilever and the other end immersed through a liquid-air interface. The diameter of the glass fiber is ~1 micrometer and length is ~100 micrometers. The intersection between the liquid interface and the fiber surface forms a circular contact line. This device has some unique features for our study of the dynamics of wetting and moving contact line (MCL). (i) With an accurate calibration, the AFM can measure the capillary force down to ~10 pN, making the determination of the contact angle θ very accurate to within 0.1°. (ii) Because θ is determined directly by the capillary force acting on the contact line, it is the most relevant contact angle to the contact angle hysteresis. (iii) By moving the fiber up and downward, one can immediately tell whether the liquid interface is pinned or not and measure the dynamic contact angle at a high sampling rate (up to 1 MHz). Such a real-time measurement of the dynamic contact angle allows us to directly study the dynamics of MCL. (iv) By using a micron-sized thin glass fiber, one can ignore the buoyancy force acting on the fiber, minimize the effect of spatial averaging over the contact line, and study the stick-slip motion of MCL with a wide dynamic range. This technique opens a door to a wide variety of important experiments for the studies of interfacial microrheology and contact line dynamics. Currently, we are using this device to measure the friction of a fluctuating contact line and the contact angle hysteresis over different fiber surfaces [Phys. Rev. E 80, 061604 (2009)].
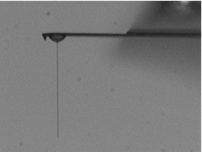